ЛАБОРАТОРНЫЕ ИСПЫТАНИЯ ПОВЫШЕНИЯ ЛЬДООБРАЗУЮЩЕЙ ЭФФЕКТИВНОСТИ КРИСТАЛЛИЗУЮЩЕГО РЕАГЕНТА АД-1 С ДОБАВКАМИ НАНОТРУБОК ОКСИДА ЦИНКА
ЛАБОРАТОРНЫЕ ИСПЫТАНИЯ ПОВЫШЕНИЯ ЛЬДООБРАЗУЮЩЕЙ ЭФФЕКТИВНОСТИ КРИСТАЛЛИЗУЮЩЕГО РЕАГЕНТА АД-1 С ДОБАВКАМИ НАНОТРУБОК ОКСИДА ЦИНКА
Аннотация
В настоящее время перераспределение влаги в нужном для человека направлении может быть осуществлено в больших масштабах путем искусственного вызывания осадков. В связи с этим ученые всего мира разрабатывают новые проекты по искусственному увеличению объема осадков. Проекты, реализуемые в разных странах, существенно отличаются друг от друга, но в большинстве из них используются пиротехнические составы, содержащие йодид серебра (AgI), которые оснащаются противоградовыми средствами. При нынешнем состоянии активных воздействий проблема разработки новых, более эффективных реагентов является актуальной. Это подтверждается тем, что средний расход противоградовых препаратов на 100 тыс. га охраняемой территории в период с 1967 по 1988 год составлял 1107 шт., а с переходом на применение противоградовых препаратов "Алазань-5" и "Алазань-6", снаряженных более эффективными составами, расход снизился до 531 шт. Перспективным направлением в вопросе повышения льдообразующей эффективности реагента является использование различных химических добавок к основному пиротехническому составу AD-1. В качестве такой добавки предлагается использовать мелкоизмельченный порошок цинка. Оксид цинка при температуре 906,2 °С начинает кипеть и превращаться в пар, что способствует образованию нанотрубок оксида цинка при сгорании топливного заряда противоградовых ракет. Образование льдообразующего аэрозоля при ракетном засеве облаков происходит при температурах от 700 до 14000 °С.
1. Introduction
The output of active ice-forming nuclei depends on many factors: composition formulation, design features of the aerosol generator; reagent dispersion conditions, etc. these factors determine the dispersity of the formed aerosol, which determines its structure and ice-forming activity. An increase in dispersity leads to an increase in the number of particles per unit mass of the reagent, however, small particles exhibit ice-forming activity at very low temperatures or under conditions of significant supersaturation of water vapor, which is not found in clouds. The formation of a large aerosol leads to the production of an aerosol active in the required temperature range (from -5 to -10 °C) without supersaturation of water vapor, but as the particles become larger, the yield from 1 gram of the composition decreases. To compensate for this, the formulation of crystallizing reagents provides for the formation of an ice-forming aerosol containing a core of combustion products, on the surface of which there are inclusions of AgI. This ensures the production of sufficiently large particles with a minimum consumption of AgI deposited on their surface. Formulations with ultra low AgI content (≤0.5%) give a spectrum of fine particles whose ice-forming efficiency is affected by dispersion conditions. An increase in the content of AgI leads to an increase in the yield of active ice-forming nuclei and an increase in the stability of their ice-forming efficiency, but this does not mean that the yield of ice-forming nuclei increases in proportion to the content of AgI , , , . Due to the fact that most active exposure projects use pyrotechnic compositions containing from 2 to 20% silver iodide, in recent years, research efforts have been directed to explore the possibilities of using the reserves of existing reagents based on AgI. The presence of such possibilities is indicated by the fact that the ice-forming efficiency of pyrotechnic compositions based on silver iodide strongly depends on the production technology of the compositions, on the dispersion of the components included in the composition, on the terms and conditions of storage of pyrotechnic products, etc. Therefore, increasing the efficiency of pyrotechnic compositions is mainly achieved by experimental selection of components for each particular pyrotechnic product. Thus, in particular, finely ground zinc powder is introduced into experimental pyrotechnic compositions, which, in combination with silver iodide, leads to the formation of ice-forming particles with a crystal lattice closer to that of ice. During the combustion of the fuel charge of anti-hail missiles, zinc oxide begins to boil and turn into steam, which contributes to the formation of zinc oxide nanoclusters. Molecular clusters of zinc oxide are polynuclear complex compounds based on a framework of zinc atoms surrounded by ligands. The choice of zinc is due to the fact that the ice-like structure of zinc, like that of AgI, promotes the adsorption of H2O molecules on its surface.
2. Materials and methods of research
Like most metals, zinc oxidizes in air, and a dense protective film of oxidized metal compounds forms on the metal surface. This film prevents the penetration of oxygen deep into the metal and thus stops further oxidation of the metal. The boiling point of zinc is 906.2 °C, and in the process of its thermal decomposition, many active atoms are formed, which create aggregates in the form of nanoclusters. Zinc interacts with water and hydrogen sulfide when the temperature rises, releasing hydrogen; and when strongly heated, it reacts with many gases: gaseous chlorine, fluorine, iodine. At temperatures below 200 ºС, in addition to zinc oxide, there is zinc peroxide ZnO2. Above 200 ºС, only ZnO is stable , . Zinc oxide nanoclusters belong to hydrophilic clusters; they can sorb the molecules of the environment on their surface, so they are surrounded by a shell. On the surface of a zinc oxide nanocluster, there can be up to several layers of H2O molecules, which leads to the formation of a surface water film with its subsequent freezing when the critical thickness is reached. In such a film, microvolumes with oriented H2O molecules are formed, which acquire an ice-like structure upon supercooling. At a sufficient size, these microvolumes serve as nuclei for ice crystals. "Multiphase" zinc oxide nanoparticles do not have standard interfaces; the concept of a phase or aggregate state is inapplicable to them. Phase diagram of the Zn–O system. The complete phase diagram of the Zn–O binary system has not been constructed. There are separate data on some parts of the phase diagram, its (T-x) cross-sections and phase transition in ZnO under the influence of external pressure . In , the (T-x)-section of the phase diagram of the Zn–O system for the condensed state was first proposed (see Fig. 1).
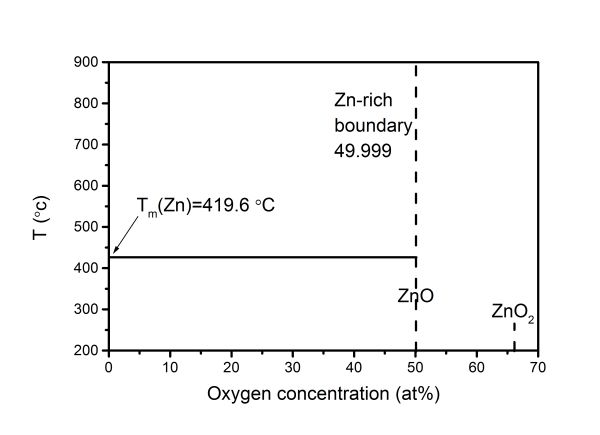
Figure 1 - (T-x)-section of the phase diagram of the binary system Zn–O at a pressure of 0.1 MPa
Note: L denotes the solubility of oxygen in Zn at different temperatures. Tm(Zn) – melting point of zinc (419.6 ºС)
ZnO(solid) ⇔ Zn(g) + 0.5 O2(g).
Simultaneously with this process, the process of sublimation proceeds:
ZnO(solid) ⇔ ZnO(g).
The study of zinc oxide evaporation by high-temperature mass spectrometry using platinum and quartz effusion chambers showed that in the temperature range of 1200-1450K, the vapor over ZnO(solid) consists of Zn atoms(g) and O2(g) molecules, while ZnO(g) molecules were found only at temperatures above 1500 K [9]. At 1540 K, the composition of the gas-vapor phase over ZnO consists of 99.94% of zinc oxide dissociation products: Zn(g) and O2(g). In the presence of an excess of zinc vapor, the evaporation rate of zinc oxide sharply increases (by a factor of 108-1010) . The authors of explain the increase in the evaporation rate of ZnO in the presence of Zn vapor by the interaction of zinc with trace amounts of H2O and CO2, which leads to the formation of H2 and CO, which reduce zinc oxide.
3. Some results of studies of ZnO nanostructures obtained in a dry and wet chamber
Previously, glass slides are installed at the bottom of the cloud chamber, which are covered with lids. A certain amount of the prototype (zinc) is placed on the graphite substrate of the reagent sublimation device. The large chamber is cooled to a predetermined temperature, and an artificial cloud environment is created in the chamber using an ultrasonic steam generator. A current of the order of 100-120 A is applied to the contacts of the graphite substrate, the substrate is heated to temperatures of 1200-2000 °C and zinc is sublimated. After that, the air in the chamber is mixed by fans. In the chamber, under the influence of high temperature, zinc interacts with oxygen to form ZnO oxide nanoparticles:
2Zn+O2=2ZnO.
Next, zinc oxide nanostructures are deposited on glass slides, which are removed from the chamber and examined under an optical and electron microscope. During the sublimation of zinc in a dry chamber, zinc oxide nanoparticles are formed in the form of nanotubes with a diameter of about 30-50 nm and a length of several microns. Figure 2 shows a hollow nanostructure obtained in a dry chamber.
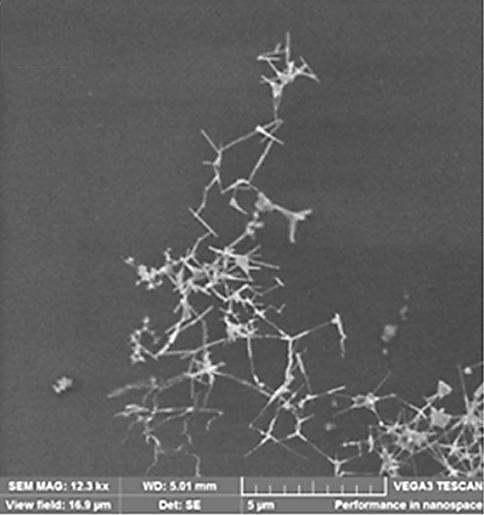
Figure 2 - Zinc oxide nanostructure obtained in a dry chamber
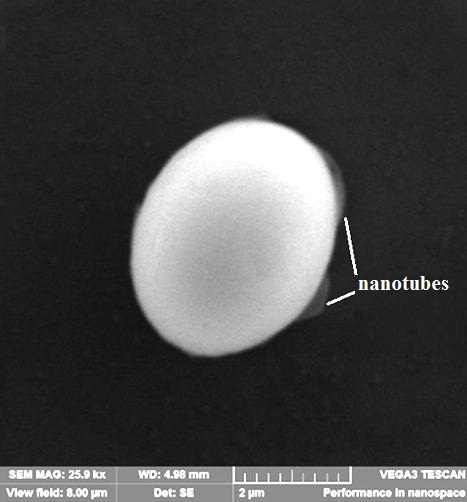
Figure 3 - Nanostructure of zinc oxide obtained in water vapor
Table 1 - Ice forming component test results AD-1 at different temperatures
Temperature, оС | Reagent AD-1, g | Specific output, g-1 |
-6.8 | 0.01 | 6.5Е11 |
-7.0 | 0.01 | 5.3Е12 |
-7.2 | 0.01 | 5.3Е12 |
-7.3 | 0.01 | 2.1Е12 |
-7.5 | 0.01 | 3.2Е12 |
-8.2 | 0.01 | 3.3Е12 |
-8.7 | 0.01 | 1.3Е12 |
-9.4 | 0.01 | 1.6Е12 |
-9.7 | 0.01 | 6.4Е12 |
-9.8 | 0.01 | 2.3Е12 |
-10.2 | 0.01 | 1.2Е12 |
-10.6 | 0.01 | 1.2Е12 |
-11.9 | 0.01 | 5.7Е12 |
-13.4 | 0.01 | 4.4Е12 |
In laboratory studies, finely dispersed zinc powder was used, which was introduced into the initial pyrotechnic composition AD-1 in relation to its total mass – 3%, 6% and 9%, respectively. It was found that the experimental reagent AD-1 with the addition of zinc in the amount of 6% of the total mass of the pyrotechnic composition has the highest activity. The test results are presented in table 2.
Table 2 - Average values of the yield of crystallization nuclei of the ice-forming component of AD-1 with the addition of zinc (6%) at different temperatures
Temperature, оС | Reagent AD-1+Zn(6%) | Specific output, g-1 |
-5.0 | 0.00052 | 1.8Е13 |
-4.2 | 0.00052 | 1.2Е13 |
-3.4 | 0.00052 | 1.0Е13 |
-2.2 | 0.00052 | 1.2Е13 |
-2.0 | 0.00052 | 4.0Е12 |
-1.6 | 0.00052 | 4.7Е12 |
-1.1 | 0.00052 | 9.0Е12 |
-1.0 | 0.00052 | 6.6Е12 |
-11.2 | 0.01 | 9.0Е13 |
-10.6 | 0.01 | 5.4Е13 |
-10.4 | 0.01 | 1.4Е14 |
-10.2 | 0.01 | 6.3Е13 |
-10.0 | 0.01 | 6.0Е13 |
-9.6 | 0.01 | 5.2Е13 |
-7.5 | 0.01 | 6.9Е13 |
Table 3 presents the results of laboratory studies of the ice-forming efficiency of the AD-1 composition with various mass concentrations of zinc.
Table 3 - Specific yield of AD-1 reagent particles with addition of zinc
t, оС | -11 | -9 | -7 | -5 |
СZn, % | Specific output, g-1 | Specific output, g-1 | Specific output, g-1 | Specific output, g-1 |
0 | 3.2 х 1012 | 2.7 х 1012 | 2.5 х 1012 | 6.5 х 1011 |
1 | 8.8 х 1012 | 7 х 1012 | 5.6 х 1012 | 1.2 х 1012 |
2 | 1.5 х 1013 | 1.2 х 1013 | 8.2 х 1012 | 4.5 х 1012 |
3 | 2.8 х 1013 | 2.2 х 1013 | 1.1 х 1013 | 7.2 х 1012 |
4 | 4.6 х 1013 | 3.6 х 1013 | 1.6 х 1013 | 9.5 х 1012 |
4.7 | 6.5 х 1013 | 5.2 х 1013 | 2.5 х 1013 | 1.1 х 1013 |
5 | 7.0 х 1013 | 5.9 х 1013 | 3.0 х 1013 | 1.3 х 1013 |
5.5 | 7,3 х1013 | 6,2 х1013 | 3,3 х1013 | 1,5 х1013 |
6 | 7.5 х 1013 | 6.3 х 1013 | 3.4 х 1013 | 1.6 х 1013 |
6.6 | 6.5 х 1013 | 5.2 х 1013 | 2.5 х 1013 | 1.4 х 1013 |
7 | 5.2 х 1013 | 4.3 х 1013 | 1.9 х 1013 | 1.0 х 1013 |
8 | 3.4 х 1013 | 3.0 х 1013 | 1.4 х 1013 | 7.2 х 1012 |
9 | 2.2 х 1013 | 1.9 х 1013 | 1.0 х 1013 | 5.3 х 1012 |
The specific yield of reagent particles depending on the concentration of zinc oxide is shown in figure 4.
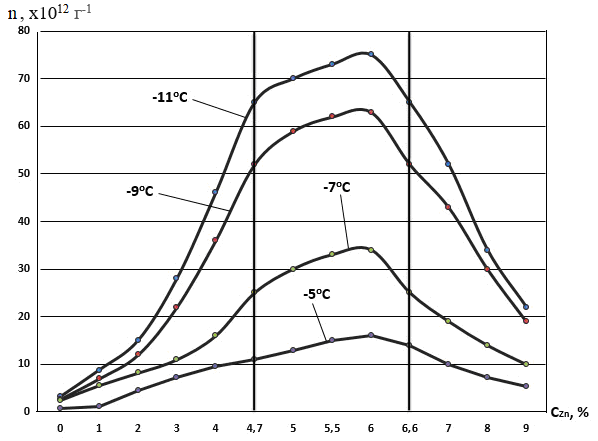
Figure 4 - Dependence of the specific yield of ice-forming particles on concentrations of zinc in pyrocomposition AD-1 for different temperature levels
4. Conclusion
The obtained results of laboratory tests showed that the presence of finely dispersed zinc powder in the composition of the initial ice-forming fuel AD-1 in relation to the total mass of the composition of 6% sharply increases the yield of ice-forming particles in the entire range of accepted temperatures. At the same time, the yield of ice-forming particles at a temperature of minus 12 оC increases by almost an order of magnitude, and in the temperature range from minus 2 оC to minus 4 оC, it almost doubles. It was found that the ice-forming properties of zinc oxide nanoclusters depend on the environmental conditions where sublimation is performed. During sublimation in a dry chamber, zinc oxide clusters with a diameter of about 30-50 nm are formed . During sublimation in a large cloud chamber in the presence of water vapor and at negative temperatures, zinc oxide clusters are formed with sizes from 50 nm to 10 μm, which have a hollow structure and consist of many nanotubes with a diameter of 1-5 nm. Nanoclusters formed during sublimation in a dry chamber do not have ice-forming properties, while nanoclusters formed during sublimation of zinc in the presence of water vapor have good ice-forming properties, with a specific yield of up to 1013 g-1. On the basis of the original ice-forming fuel AD-1, a method has been developed for obtaining an effective pyrotechnic composition for equipping meteorological rockets designed for active impact on thunderclouds in order to artificially increase liquid precipitation and combat hail. This composition can also be used in the creation of promising ground-based generators for active influence on supercooled fogs in order to provide favorable meteorological conditions for the operation of spaceports, airports and road communications.