DETECTING DUST-CONTAMINATED SNOW COVER BY EPR SPECTROSCOPY
Тентюков М.П.
Кандидат географических наук, доцент, Институт биологии Коми НЦ УрО РАН
ВЫЯВЛЕНИЕ ПЫЛЕВОГО ЗАГРЯЗНЕНИЯ СНЕЖНОГО ПОКРОВА С ПОМОЩЬЮ ЭПР-СПЕКТРОСКОПИИ
АннотацияПредложен новый способ определения запыленности снежного покрова, основанный на регистрировании ослабления ультрафиолетового (УФ) компонента солнечного излучения в снежной толще путем ЭПР-регистрации в оксиде магния ЭПР-сигнала линий Mn2+, легко детектируемый в спектре ЭПР по секстету линий Mn2+, и интенсивность которых индуцирована фотокаталитической активностью MgO. На основе данного свойства оксида магния разработан новый способ оценки запыленности снежного покрова, предусматривающий экспонировании высокодисперсного порошка оксида магния в снежной толще и измерение амплитуды характерного ЭПР-сигнала ионов марганца. Апробация способа при сравнительной оценке запыленности снега на двух ключевых участках, один из которых расположен в поле, а другой возле автомобильной дороги, показала, что с глубиной градиент ослабления ЭПР-сигнала на первом участке меньше, чем на втором. Данный способ может быть положен в основу ЭПР-детектирования УФ-прозрачности снежного покрова, что расширяет спектр методик, предназначенных для исследований снежного покрова.
Ключевые слова: Ионы переходных металлов, оптическая прозрачность снега, снежный покров, солнечная радиация, ультрафиолетовое излучение, электронно-парамагнитный резонанс.
Mikhail P.T.
Senior Staff Scientist, Docent, Institute of Biology, Komi Science Centre, Ural Branch, Russian Academy of Sciences
DETECTING DUST-CONTAMINATED SNOW COVER BY EPR SPECTROSCOPY
AbstractA new approach to detecting dust-contaminated snow is proposed, which is based on estimating the attenuation of the ultraviolet (UV) component of solar radiation probed by magnesium oxide (MgO) powder exposed in a snow cover. The presence of contamination is readily detected by monitoring the attenuation of a sextet of Mn2+ impurity ion lines in the electron paramagnetic resonance (EPR) spectrum of powder, the intensity of which is determined by the photocatalytic activity of MgO. Based on this property of MgO, a new method of estimating the dust contamination of snow is developed, according to which fine MgO powder is exposed in the depth of snow and the gradient of attenuation of the characteristic EPR signal of impurity manganese ions is measured. The proposed method was verified by comparative evaluation of the dust contamination of snow cover in two sites, one of which was situated in an open field and the other, near a motor highway. The results showed that the gradient of EPR signal attenuation in the depth of snow in the first site is lower than that in the second case. The proposed method can provide a basis for EPR monitoring of the UV transparency of snow, which expands the arsenal of tools intended for the characterization of snow cover.
Keywords: Transition metal ions; Optical transparency of snow; Snow cover; Solar radiation; Ultraviolet radiation; Electron paramagnetic resonance
- Introduction
The content of aerosols in the Earth’s atmosphere is continuously increasing (Ivlev et al., 2011), which is determined to a considerable degree by enhanced contamination of the atmosphere through emission of dust particles from the Earth’s surface. During the past century, the amount of dust in the troposphere has almost doubled (Mahowald et al., 2010) and the annual supply of dust to the gaseous shell of our planet reaches 1600 billion tons (Lisitsyn et al., 2011). Under these conditions, the deposition of snow is accompanied by the accumulation of dusty aerosol particles in the snow cover. In order to detect dust in the snow cover, the samples of snow are conventionally taken and melted, the melt-water is filtered, and the filtrate is weighed. The presence or absence of contamination is judged by comparison of the weight of filtrates obtained from melt-water of snow samples taken in background (reference) and dust-contaminated sites (Glazovskii et al., 1983). According to another method (Elpat’evskii, 1978), the level of snow cover contamination is evaluated by comparing the degree of mineraliization of melt-water obtained from snow samples taken in background (reference) and contaminated sites. The dust contamination of snow can also be detected by measuring the electric conductivity of snow (Makarov, 2007).
As is known, the optical transparency of snow cover varies depending on the dust content (Kuz’min, 1957). The transparency of snow is conventionally measured using instruments (pyrheliometers, pyranometers, photometers, photocells) based on the thermoelectric, photoelectric, and photometric principles. Differences between these methods and related equipment, the spectral sensitivity of which extends over a very broad wavelength range (from 350 to 830 nm), make the results of these measurements hardly comparable (Kuz’min, 1957, p. 137) and hinder evaluation of the degree of snow contamination by dust based on the results of measurements of the optical transparency of snow cover. The situation is additionally complicated by the fact that upper and lower snow layers differently absorb and scatter solar radiation in various spectral intervals (Dozier et al., 2009; Middleton, 1952; Warren et al., 1980; Picard et al., 2009). The introduction of necessary corrections requires carrying out complicated model calculations (Bohren and Barkstrom, 1974; Kaempfer et al., 2007), which are not always properly justified, since any model must be verified based on experimental field data. Instead, the procedure of field verification is frequently replaced by laboratory experiments and simulations (Salm, 2004). Apparently, the implementation of a justified and commonly accepted snow transparency–dust content relationship into monitoring of the snow cover contamination is also hindered by the lack of simple dust detectors, while the use of available instruments is hindered by complexity of their use under field conditions.
The present article describes the method and presents results of the EPR evaluation of the optical transparency of snow in the UV spectral range, which can be used for detecting the dust contamination of a snow cover.
- Justification of the Proposed Method
It is proposed to monitor the optical transparency of a snow cover by using an EPR marker in the form of a highly dispersed (with average grain size below 100 nm) magnesium oxide (MgO) powder, also containing impurity metal ions with mixed valence (Mn2+/3+, Fe2+/3+, Cr2+/3+). It has been established previously (Tentyukov and Lyutoev, 2008) that changes in the intensity of EPR lines of these ions are induced by the photocatalytic activity of MgO. Accordingly, it is suggested that the appearance of Mn2+ ions upon the exposure of MgO powder under atmospheric conditions is related to the photocatalytic oxidation of impurities in the dispersed marker, which makes it most informative for solving the task. The EPR signal of manganese ions is readily detected by a sextet of Mn2+ ion lines (Vlasova et al., 1987; Volodin, 1992). These lines are due to valence transitions in the impurity manganese ions (Mn3+/2+) (Chiesa et al., 2010; Valia and Rooney, 2000; Wu et al., 2009). The spectral sensitivity of Mn2+ ions in MgO crystals is related to the UV component of solar radiation. The spectral interval of this radiation absorption by MgO falls within 180—200 nm (hard “vacuum” UV range) (Zamaraev and Parmon, 1996). At the same time, it was also established (Roessler and Walker, 1967; Zakharenko, 1997) that MgO upon long-term exposure in air exhibits optical absorption at 300—400 nm in the near-UV range. This fact is yet not explained and seems paradoxical. However, taking into account the hygroscopicity of magnesium oxide, it can be suggested that that near-UV radiation quanta are absorbed by a boundary medium rather than by the MgO crystals. This medium is formed as a result of MgO interaction with a liquid film into which metal ions with mixed valence enter in a soluble form. When magnesium oxide is exposed in air, this liquid film is formed due to water vapor condensation on the surface of mineral grains. This film possesses photosensitizing properties, which favor a decrease in the bandgap width and hence, an increase in the UV radiation absorption.
A possible shift of the spectral interval of UV absorption by magnesium oxide exposed under real atmospheric conditions was demonstrated in experiments (Zakharenko and Daibova, 2011; Zakharenko and Parmon, 1999; Parmon and Zakharenko, 2006). These results show that reactions of the photoelectrocatalytic oxidation of impurities on the surface of MgO crystals irradiated by daylight at relatively low temperatures are quite probable (Zakharenko and Parmon, 2000). It is possible that the aforementioned liquid film first appears on local nanosized active surface regions of MgO crystals. According to Cort and Scot (2001), the presence of such regions significantly increases the intensity of heterogeneous surface chemical reactions. It is suggested that these active nanosized regions may be considered as important precursors initiating the onset of formation of a new boundary medium characterized by certain electrohydrolytic and photosensitizing properties. The appearance of these properties shifts the photosensitizing activity of the liquid film toward the near-UV spectral interval (300—400 nm), which makes the photoelectrocatalytic oxidation of impurities on the surface of MgO crystals possible under daylight illumination at relatively low temperatures. As a result, the boundary medium changes the valence state of impurity metal ions, which is manifested by the aforementioned EPR effect.
- Experimental Part
Figure 1a shows the general view of a probing device for the exposure of highly dispersed magnesium oxide in a snow cover. The device comprises a stem-holder (1) with fixing holes (2), in which tightly closed silica glass tubes (3) containing magnesium oxide powder are mounted. The experiments were performed with highly dispersed (100—500 nm) reagent-grade MgO powder calibrated with respect to the hygroscopic moisture content, which was achieved by preliminary drying at 95°С for 30 min. After drying, the powder was charged into silica glass tubes with a diameter matched with that of a hole in the microwave resonator of an EPR spectrometer. This circumstance allowed the transportation, exposure, and EPR spectroscopy of MgO powder to be carried out in the same tube without extraction. The height of powder filling in the tubes was ~5 mm and the sample powder weight was within 1.8—2.2 g.
The device with powder-charged tubes was set on a snow-covered field in a green suburb zone near Syktyvkar. In order to mount the tubes at certain depths of snowpack in accordance with its structure and the monitoring program, the stem-holder is equipped with movable sliders. The holder with tubes was inserted into snow so that the upper (reference) tube was situated at a height of 5 cm above the snow surface (Fig. 1b). This distance was maintained constant to the end of experiment (Fig. 1c).
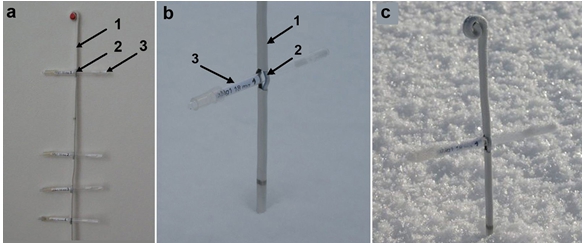
Fig. 1 - (a) General view of the probing device and (b, c) examples of its arrangement in the snow cover (see text for explanations)
The device was exposed for six days (January 12 to 17, 2011). Variation of the magnitude of the characteristic EPR signal caused by the attenuation of solar radiation at various depths of snow was monitored by measuring the height of manganese ion lines. Analysis of the exposure-induced variation of the height of Mn2+ impurity ion lines in the EPR spectrum showed that the intensity () of the Mn2+ signal from tubes situated at various depths was significantly different (Fig. 2). The results indicated that, due to the scattering of solar radiation in depth of the snow cover, the light-induced EPR effect in MgO powder in the tubes situated at various depths decreases with increasing snow layer thickness. Evidently, under otherwise equal conditions, the light penetrating in depth of a dust-contaminated snow will be even more significantly scattered. Therefore, the EPR effect can be used to reveal dust contamination of a snow cover.
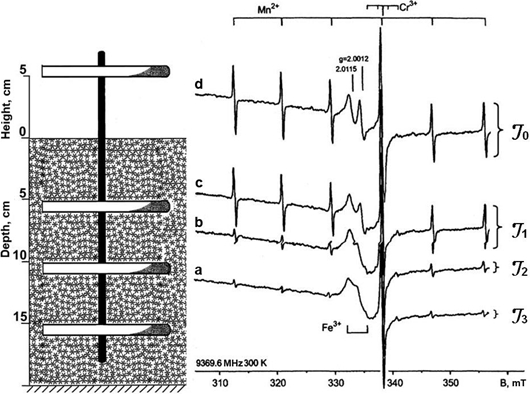
Fig. 2 - Schematic diagram of tubes with MgO powder situated in various positions relative to the snow surface and the EPR spectra measured upon exposure of these tubes (see text for explanations)
For experimental verification of the proposed method, the optical transparency of snow cover was studied by EPR in two sites, one of which (Highway) was situated near the Syktyvkar—Ukhta motor highway, while the other (Field) was situated in an open field in a green zone 4 km westward from Syktyvkar. The tubes with MgO powder samples were exposed for six days (January 20 to 26, 2011). The levels of dust contamination in the Field and Highway sites were compared by measuring the content of insoluble fraction in melt-water samples obtained from the upper 18-cm-thick layer of snow in these sites. The obtained melt-water (six samples from each site) was filtered (18-cm sol-free paper filters, white-ribbon grade) and the filtrate was dried and weighed to within 0.1 mg on an electronic balance. The solid residue weight was 2.1 ± 0.3 mg/L in the Field site and 16.8 ± 3.3 mg/L in the Highway site. The confidence interval was calculated for α = 0.95. The relative error of measurements was within 10—20%.
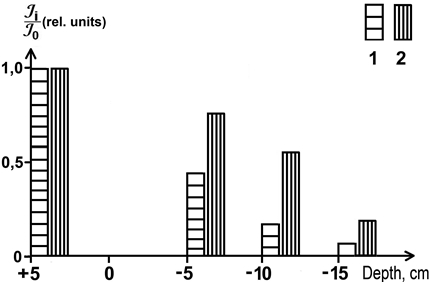
Fig. 3 - Variation of the characteristic EPR signal intensity from MgO powder exposed in tubes situated at various depths of snow in (1) Highway and (2) Field sites (normalized to maximum of the signal observed for MgO powder in the uppermost tube exposed to direct sunlight 5 cm above the snow surface)
Analysis of the EPR spectra showed that the intensity of Mn2+ lines decreases with increasing depth in the snow. Figure 3 shows variation of the EPR signal amplitude caused by the attenuation of solar radiation penetrating to various depths in the snow cover (relative to maximum of the signal observed for MgO powder in the uppermost tube exposed to direct sunlight). The relative signal intensity was calculated as the ratio
, where
is the EPR signal intensity observed for a tube exposed at the ith depth level and
is the signal intensity observed for the uppermost tube exposed to direct sunlight. As can be seen from Fig. 3, the signal intensity decay in the depth of snow is more pronounced in the Highway site (histogram 1), which is apparently due to the presence of dust contamination caused by intense road traffic. The signal intensity decay in the Field site is much less pronounced (histogram 2). Therefore, the obtained results show that the EPR monitoring of the transparency of snow by measuring the intensity of a signal arising in a highly dispersed MgO powder exposed in depth of snow can be used for detecting dusty aerosol contamination of a snow cover.
- Conclusion
A new approach to detecting dust-contaminated snow is proposed, which is based on estimating attenuation of the ultraviolet (UV) component of solar radiation in the depth of snow cover. The attenuation of solar radiation is probed by magnesium oxide (MgO) particles exposed in a dusty snow cover and detected by monitoring a sextet of Mn2+ impurity ion lines in the EPR spectrum. It has been experimentally shown that the gradient of attenuation of the EPR line intensity in the spectra of samples exposed at various depth of snow is determined by the photocatalytic activity of MgO. Based on this EPR effect, a new method of EPR monitoring of the UV transparency of snow cover can be developed, which would expand the arsenal of tools intended for the characterization of snow cover.
The proposed method can be used to develop simple EPR detectors, representing a new class of instruments for determining the optical transparency of snow, which will supplement the existing set of multispectral sensors (pyrheliometers, pyranometers, photometers, photocells) based on the thermoelectric, photoelectric, and photometric principles. The use of multispectral sensors (mostly intended to measure the albedo of snow) for detecting the presence of dust is low informative, since it has been established that solar radiation is differently reflected from a dusty snow surface in the visible spectral range (Roessler and Walker, 1952; Warren and Warren, 1980). Under these conditions, the EPR monitoring of the optical transparency of a snow cover can be more adequate than the use of multispectral sensors. In addition, the EPR detectors can be used to calibrate the aforementioned instruments for the investigation of snow transparency by comparison to calculations of the extinction of a snow cover. However, this hypothesis requires verification with the use of additional equipment.
Acknowledgments
The author is grateful to Dr. V. P. Lyutoev (Institute of Geology, Komi Scientific Center, Syktyvkar) for conducting EPR measurements and interpreting Mn2+impurity ion lines in the spectra of magnesium oxide powder.
References
- Vlasova, M.V., Kakazei, N.G., Kalinichenko, A.M., Litovchenko A.S., 1987, Radiospectroscopic Properties of Inorganic Materials, Naukova dumka, Kiev [in Russian].
- Volodin, A.M., 1992, EPR study of the mechanism of active center formation on the surface of MgO in the presence of N2O and O2 molecules, Zh. Khim. Fiz. 11 (8), 1054-1063.
- Glazovskii N.F., Zlobina, A.I., Uchvatov, V.P., 1983, Chemical composition of snow cover in some regions of Verkhneokskii basin (Regional ecology monitoring), Nauka, Moscow, 67-86 [in Russian.
- Elpat’evskii, P.V., 1976, Chemical composition of snow melt-water and its change under the action of technogenic factors: Geochemistry of hypergenesis zone and human technical activity, Vladivostok, 45-63 [in Russian].
- Zamaraev, K.I., Parmon, V.N., 1996, Heterogeneous catalysis and photoadsorption in troposphere: possible action upon the global chemistry of the Earth’s crust, Kinetika i kataliz 37, 732-738.
- Zakharenko, V.S., Daibova,E.B., 2011, Photochemical activity of deposited aerosol obtained from periclase mineral crystal (MgO) in ambient air, Optika atmosfery i okeana 24 (6), 516-520.
- Zakharenko, V.S., Parmon V.N., 1999, Composition of adsorbed layer on magnesium oxide surface formed under the action of ambient air, Zh. Fiz. Khim. 73 (1), 124-127.
- Zakharenko, V.S., Parmon, V.N., 2000, Photoadsorption and photocatalytic processes affecting the Earth’s atmosphere: II. Dark and photostimulated adsorption of freon (CHF2Cl) on MgO, Kinetika i kataliz 41 (6), 834-838.
- Ivlev, L.S., 2011, Aerosol effect on climate processes, Optika atmosfery i okeana 24 (5), 392-410.
- Kuz’min, P.P., 1957, Physical Properties of Snow Cover, Gidrometeoizdat, Leningrad [in Russian].
- Lisitsyn, A.P., 2011, Aride sedimentation in world ocean: Scattered atmospheric deposit substance, Geologiya i geofizika 52 (10), 1398-1439.
- Makarov V.N., 2006, Geochemical Monitoring of Atmospheric Deposits in Central Yakutia, Institute of Permafrostology SO RAN, Yakutsk [in Russian].
- Parmon, V.N., Zakharenko, V.S., 2006, Photocatalysis and Photoadsorption on Atmosferic Aerosols: Aerosols in Siberia, ed. by K.P. Kutsenogii, SO RAN, Novosibirsk, 299-327 [in Russian].
- Tentyukov, M.P., Lyutoev, V.P., 2008, EPR spectroscopy of dry aerosols, Optika atmosfery i okeana 21 (9), 789-792.
- Bohren, C. F., Barkstrom, B.R., 1974, Theory of the optical properties of snow, Journal of Geophysical Research 79 (30), 4527-4535; doi: 10.1029/JC079i030p04527.
- Chiesa, M., Paganini, M.C., Giamello, E., 2010, EPR of charge carries stabilized at the surface of metal oxides, Applied Magnetic Resonance 37, 605-618.
- Cort, A., Scot, T.M., 2001, Atmospheric nanoparticles, Reviews in Mineralogy and Geochemistry 44 (1), 293-349; doi:10.2138/rmg.2001.44.08.
- Dozier, J., Green, R.O., Nolin, A.W., Painter, T.H., 2009, Interpretation of snow properties from imaging spectrometry, Remote Sensing of Environment 113, S25-S37; doi:10.1016/j.rse.2007.07029.
- Kaempfer, T.U., Hopkins, M.A., Petrovich, D.K., 2007, A three-dimensional microstructure-based photon-tracking model of radiative transfer in snow, Journal of Geophysical Research 112, D24; doi: 10.1029/2006JD008239.
- Mahowald, N.M., Kloster, S., Engelstaedter, S., et al., 2010, Observed 20th century desert dust variability: impact on climate and biogeochemistry, Atmospheric Chemistry and Physics 10, 10875-10893; doi:10.5194/acp-10-10875-2010.
- Middleton, W.E., Mungall, A.G., 1952, The luminous directional reflectance of snow, Journal of the Optical Society of America, 42, 572-579.
- Picard, G., Arnaud, F., Domine, F., Fily M. 2009, Determining snow specific surface area from near-infrared reflectance measurements: Numerical study of the influence of grain shape, Cold Regions Science and Technology 56(1), 10-17; doi: 10.1016/j.coldregions.2008.10.001
- Roessler, D.M., Walker, W.C., 1967, Electronic spectrum and ultraviolet optical properties of crystalline MgO, Physical Revue 159 (3), 733-738.
- Salm, B., 2004, A short and personal history of snow avalanche dynamics, Cold Regions Science and Technology, 39 (2-3), 83-92.
- Valia, A.-E., Rooney, J.J. 2000, A novel ESR method based on dilute solid solution of Mn3+/ Mn2+ ions in MgO for detecting spillover of hydrogen from noble metals, Journal of Molecular Catalysis A: Chemical 159, 429-432.
- Warren, S.G., Warren J.W., 1980, A model for the spectral albedo of snow. II: Snow containing atmospheric aerosols, Journal of Atmospheric Science 37, 2734-2745; doi: http://dx.doi.org/10.1175/1520-0469(1980)037<2734:AMFTSA>2.0.CO;2.
- Wu, X.-X., Fang, W., Feng W.-L., Zheng W.-C., 2009, Study of EPR parameters and defect structure for two tetragonal impurity centers in MgO:Cr3+ and MgO:Mn4+ crystals, Applied Magnetic Resonance 35, 503-510.
- Zakharenko, V.S., 1997, Photoadsorption and photocatalytic oxidation on the metal oxides - components of tropospheric solid aerosol under the Earth's atmospheric conditions, Catalysis Today 39, 243-246.