ФОТОВОССТАНОВЛЕНИЕ ДРОЖЖЕВЫХ КЛЕТОК, ОБЛУЧЕННЫХ УЛЬТРАФИОЛЕТОВЫМ B-ИЗЛУЧЕНИЕМ, ИНДУЦИРОВАННОЕ КРАСНЫМ СВЕТОМ
ФОТОВОССТАНОВЛЕНИЕ ДРОЖЖЕВЫХ КЛЕТОК, ОБЛУЧЕННЫХ УЛЬТРАФИОЛЕТОВЫМ B-ИЗЛУЧЕНИЕМ, ИНДУЦИРОВАННОЕ КРАСНЫМ СВЕТОМ
Аннотация
Установлена эффективность длинноволнового видимого света в восстановлении облученных ультрафиолетом В (UVB, 313 нм) клеток (Saccharomyces cerevisiae). Спектр действия фотовосстановления имеет основной оптический максимум в красной области около 680 нм, и наблюдаемый процесс принципиально отличается от известных процессов ферментативной фотореактивации, которые индуцируются только ультрафиолетовым (320-400 нм)/синим (400-450 нм) светом. Индуцированное красным светом фотовосстановление клеток не зависит от температуры в течение кратковременного (несколько минут) периода облучения, что свидетельствует о том, что на первичной световой стадии процесса происходит фотохимическая реакция. Предполагается, что фитохромоподобный фоторецептор с билиновым хромофором может выступать в качестве сенсора красного света для процесса фотовосстановления в дрожжах, повышая устойчивость клеток к УФ-излучению.
1. Introduction
UVB radiation (290–320 nm) is known to be the component of sunlight mainly responsible for cytotoxic, mutagenic, and carcinogenic effects on living systems , . The major contribution to the damaging processes is made by cyclobutane pyrimidine dimers, which are primarily formed as a result of the direct absorption of the UVB photons by DNA bases. The resistance of biological systems to the damaging effects of solar UVB radiation is provided with a variety of cellular defense and DNA repair mechanisms. Among them, light-induced processes of cell protection against photodamage play a special role . Enzymatic photoreactivation is the most widespread process, which results in the reversibility of the above-mentioned damaging effects of UVB as well as not environmental UVC (220-290 nm) radiation under further exposure to the UVA/ blue light (320-450 nm). The photoreactivation is based on the repair of the pyrimidine dimers of DNA and occurs with the participation of the light-sensitive enzymes, photolyases, with the catalytically active flavin chromophore , , . The effect of photoprotection of yeast cells against UVC (B) radiation lethality is also known . The mechanism of the photoprotection is related to UVA (320-380 nm) activation of the enzymatic synthesis of serotonin capable of binding to the DNA by intercalation and preventing generation of pyrimidine dimers. The comparison of photoprotection by serotonin and enzymatic photoreactivation indicates that their spectral sensitivity is limited by UVA and short-wave visible light. This work is aimed at studying the photorecovery activity of the long-wave visible light under exposure of yeast cells to the UVB radiation.
2. Research methods and principles
Yeast cells Saccharomyces cerevisiae were cultivated and prepared for experiments according to a standard procedure . To remove growth medium before irradiation, the 7 h log-phase cells were washed twice (1500g x 5 min) with distilled water. Cell suspensions (105 ml-1) in a mineral medium were irradiated at 220C, with stirring applied continuously. A 1000 W high-pressure mercury lamp was a source of light. A diffraction-grating spectrograph with linear dispersion of 1 nm mm-1 was used to obtain monochromatic UVB (313 nm) radiation as well as monochromatic light in the range 365–730 nm. The fluence rate of monochromatic light at each wavelength (365, 405, 436, 498, 546, 578, 610, 630, 660, 680, 710, and 730 nm) at the sample position was about 0.2 Wm-2. The fluence rate of UVB (313 nm) radiation at the sample position was 5 Wm-2. After illumination, yeast cells were placed on agar and incubated at 320C for 24 h. Survival was determined by counting microcolonies. All experiments were performed in triplicate. Deviations in determining yeast survival by microcolonies method did not exceed ±5%. Symbols on figures represent average values.
3. Main results
It is well known that S. cerevisiae cells are capable of enzymatic photoreactivation, with a maximum in the action spectrum at 365-385 nm. In other words, the viability of the UVC-inactivated cells can be recovered by subsequent irradiation with UVA in the range 350-400 nm. Using low-fluence rate monochromatic light of the visible spectrum region (600-730 nm), we revealed the effect of photorecovery of the viability of S. cerevisiae cells inactivated by UVB radiation (313 nm). Figure 1 shows a typical curve of cell photorecovery induced by monochromatic light at 680 nm (this wavelength produced the maximal effect). As seen, the maximal level of photorecovery is observed upon short-term irradiation (minutes) with low fluences of monochromatic light. The increase in the irradiation time (fluence) decreased the efficiency of photorecovery.
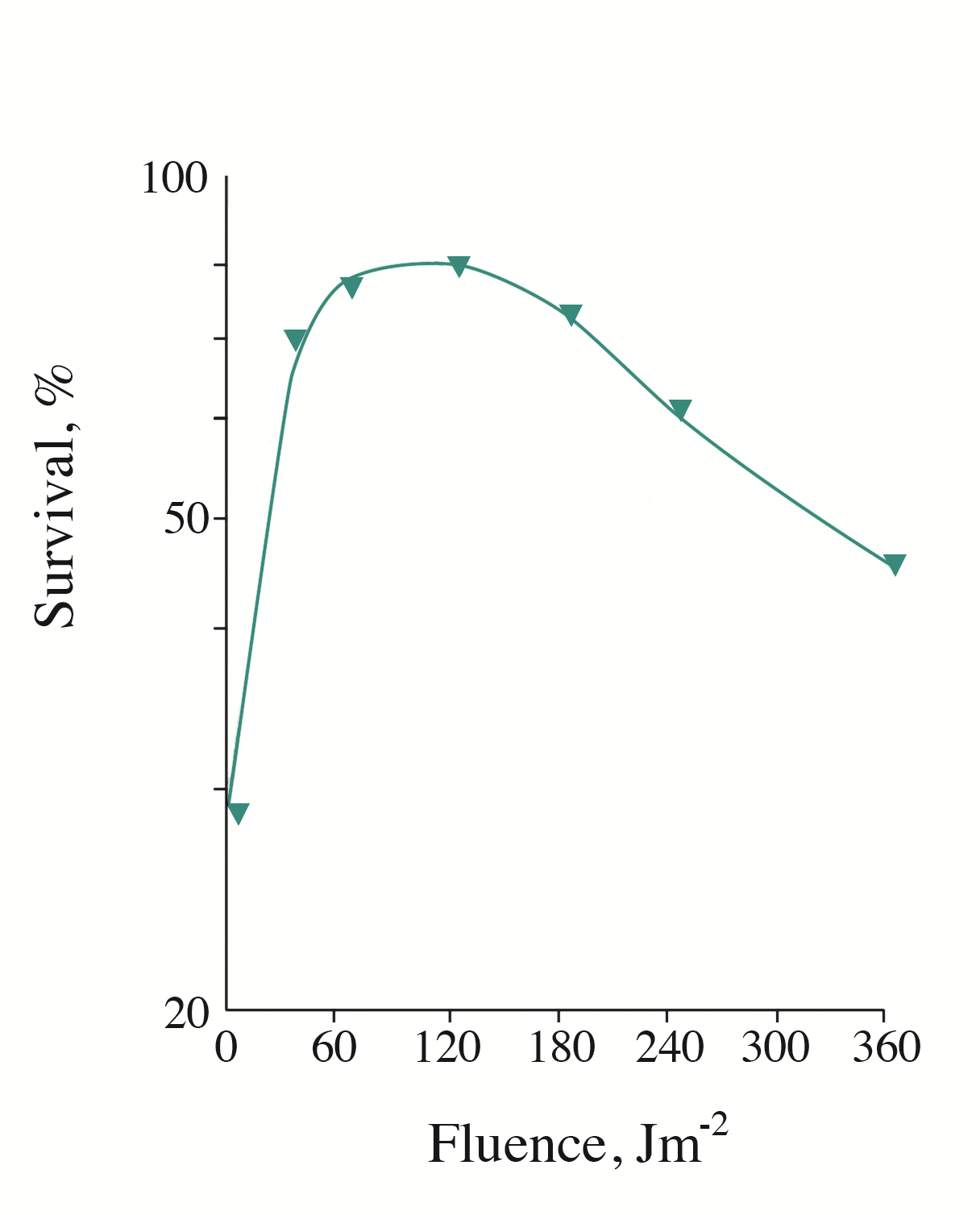
Figure 1 - Survival of UVB-inactivated cells S. cerevisiae as a function of the irradiation fluence with monochromatic light (680 nm)
Note: the UVB (313 nm) irradiation fluence (at 30% survival) was 2.1 kJm-2
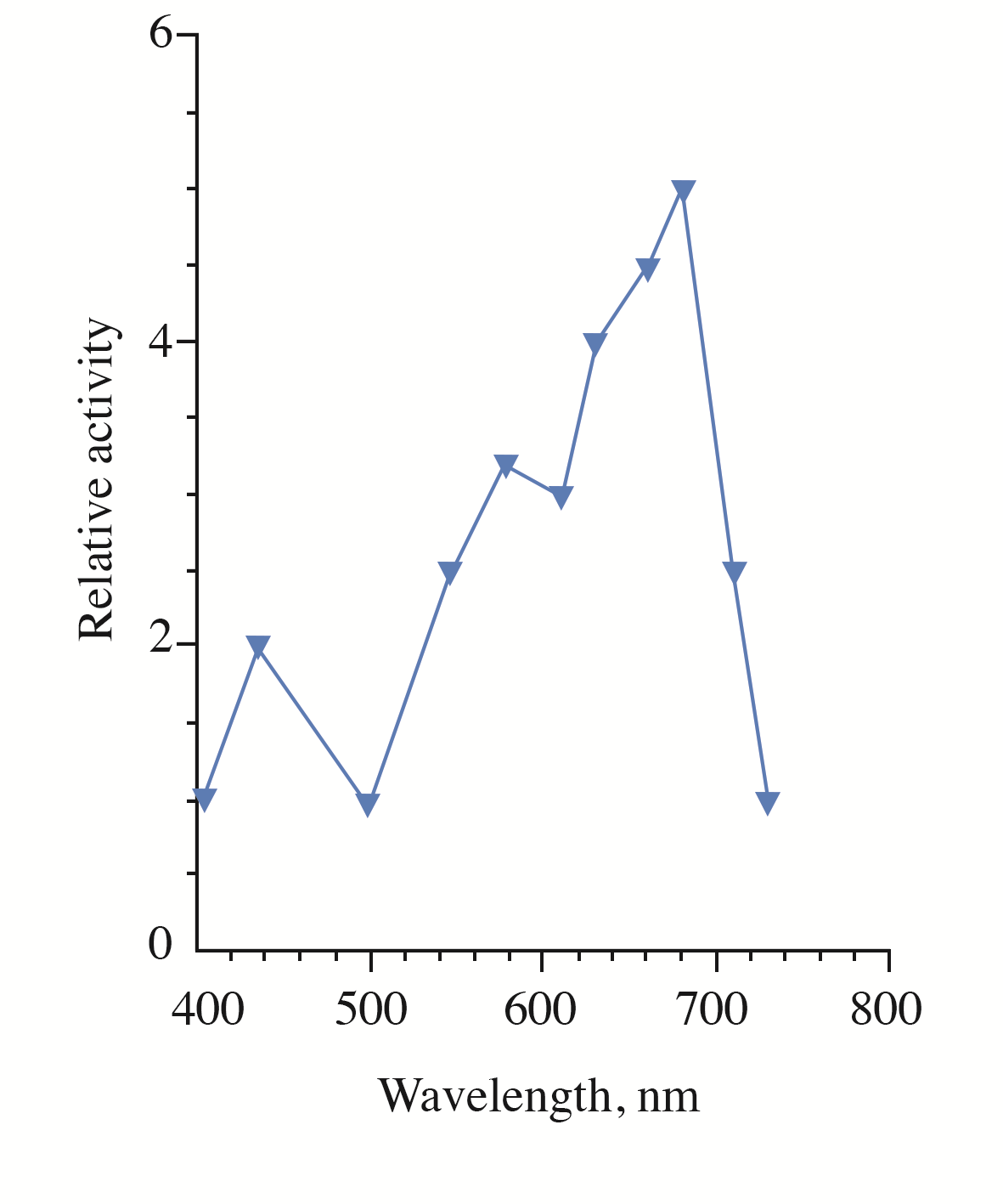
Figure 2 - The action spectrum for the photorecovery of UVB-inactivated cells S. cerevisiae
Note: relative activity indicates the efficiency of photorecovery at a certain wavelength in reference to the efficiency at 405 nm (taken as 1). The efficiency of photorecovery at each wavelength in the range 400–750 nm was determined as a reciprocal value of the monochromatic light fluence that increased the survival of the UVB-irradiated cells up to 50%
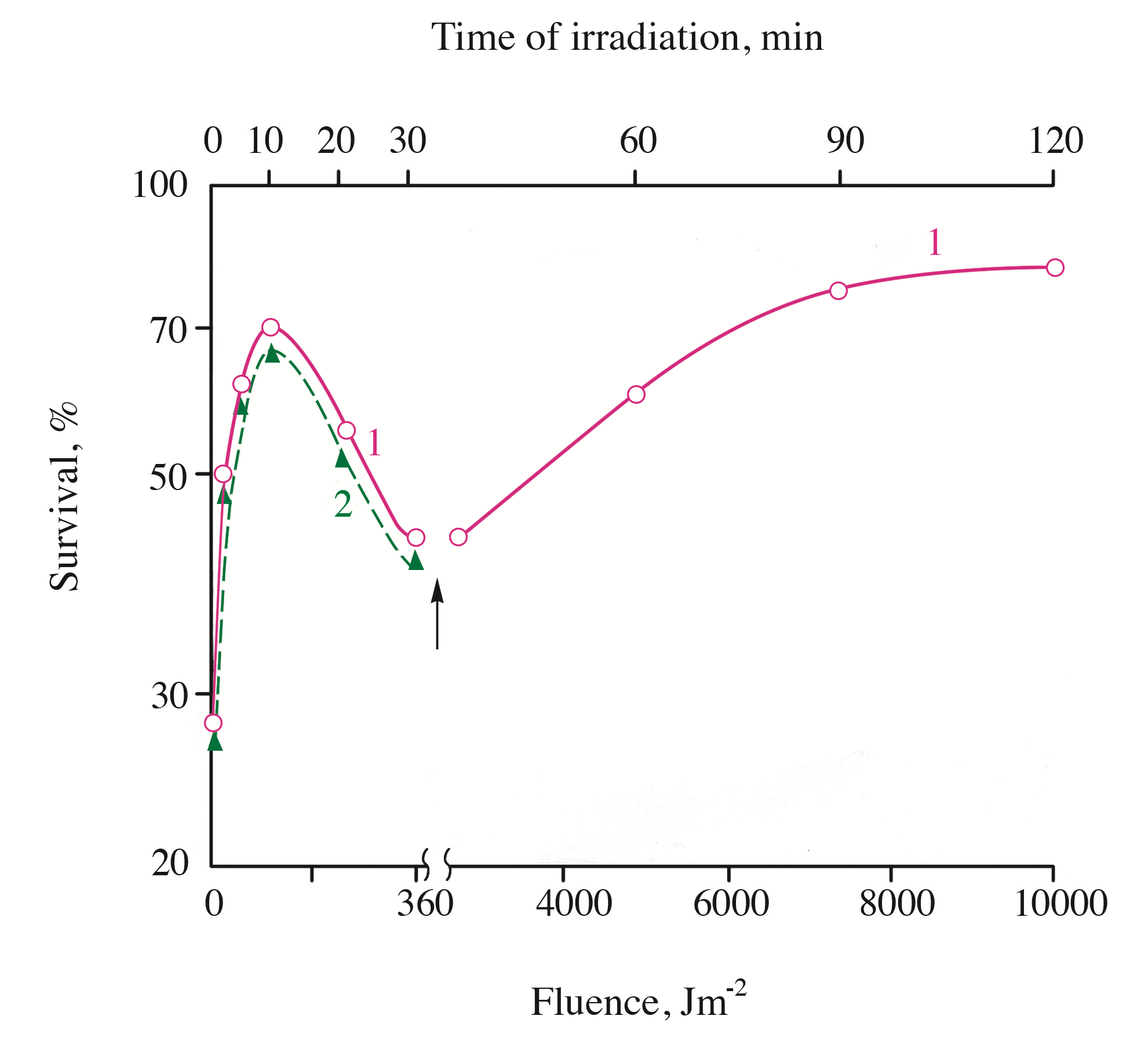
Figure 3 - Survival of UVB-inactivated cells S. cerevisiae as a function of the irradiation fluence with monochromatic light (405 nm)
Note: the cells were irradiated with monochromatic light at 220C (curve 1) or at 40C (curve 2). The arrow indicates the change of 405 nm fluence rate from 0.2 to 1.5 Wm-2. The UVB irradiation fluence (at 30% survival) was 2.1 kJm-2
4. Conclusion
We hypothesize that a phytochrome-like photoreceptor with bilin chromophore could act as a red-light sensor for the photorecovery process in yeast. Originally, phytochromes were thought to be restricted to photosynthetic organisms, but they have been later discovered in heterotrophic bacteria , and fungi . Interestingly, it was shown that the yeast Pichia pastoris synthesized a phytochrome chromophore, which could assemble with recombinant apophytochrome to form a photoactive holophytochrome. Both in vivo and in vitro difference spectra of these species with positive maximum at 660 nm were very similar to those of higher plant phytochrome A, supporting the conclusion that this phytochromic species possess a phytochromobilin chromophore. The maximum at 660 nm is slightly different from the main maximum (at 680 nm) in the action spectrum for the yeast photorecovery obtained in our work (see Figure 2). A cause of the discrepancy is presently unknown. Further genetic, biochemical and spectroscopic studies are needed to identify the yeast photoreceptor and determine the structure of its chromophore. Elucidation of the molecular mechanisms underlying the involvement of the photoreceptor in the photorecovery process is another task of further investigations.